NewsSep | 26 | 2023
How A New Grant Will Support the Quest for a More Complete Map of the Brain


Remember the days before smartphone maps, when someone would give you verbal directions for how to drive from point A to point B?
While these directions may have hit on all the main roads you needed to take to reach your destination, chances are they left out some small detail that would lead you to miss a side street or take a wrong turn.
In a way, there’s a similar challenge taking place in our quest to map the networks in the brain.
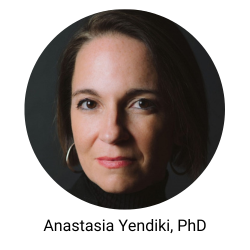
Each brain cell, or neuron, has long projections called fibers that connect to other neurons in the brain. These connections are crucial to the systems that power our cognitive and motor functioning.
Using advanced imaging technologies, researchers been able to identify some of the major highways in the brain that these signals travel along. But the side roads—those smaller connections that can’t be visualized yet—remain a mystery.
A better understanding of those smaller pathways could improve the accuracy of deep brain stimulation treatments for neurological disorders like Parkinson’s disease and psychiatric disorders like obsessive-compulsive disorder. It could also help to make brain surgery more precise and effective.Anastasia Yendiki, PhD, an investigator in the Athinoula A. Martinos Center for Biomedical Imaging at Massachusetts General Hospital and an associate professor of Radiology at Harvard Medical School, is leading a new project that aims to use advanced imaging technologies to provide a more complete map of the network of fiber pathways in the brain.
The five-year, $23.5M project is one of 11 new awards supported by the National Institutes of Health through the Brain Research Through Advancing Innovative Neurotechnologies® (BRAIN) Initiative.
Imaging Brain Connections in Unprecedented Detail
Mass General is the lead site for Yendiki’s project, BRAIN CONNECTS: The center for Large-scale Imaging of Neural Circuits (LINC). Her co-principal investigators are Suzanne Haber, PhD, at the University of Rochester and Elizabeth Hillman, PhD, at Columbia University. The project involves collaborators at five additional sites.
The goal is to produce the most detailed map yet of the axonal projections between different parts of the brain, Yendiki explains.
To accomplish this, the team will be using a brand new, state-of-the-art MRI scanner called Connectome 2.0 that is only available at the Martinos Center and was just installed this summer, as part of a separate BRAIN Initiative project led by Susie Huang, MD, PhD.
The scanner is optimized for diffusion-weighted MRI, an imaging technique that makes it possible to view these axonal projections in the brain non-invasively.
Yendiki, Huang, and colleagues at the Martinos Center will use the new scanner to conduct extensive scans of post-mortem brains. The brains will then undergo advanced microscopy, using techniques developed by other investigators in the project. This involves imaging the entire brain with a high-energy X-ray source, or slicing and processing the tissue and examining it under high-resolution microscopes.
After all the MRI and microscopy data have been collected, the team will work on developing new machine learning models to help in analyzing the results and creating a more accurate “ground truth” map of the fiber highways and byways in the brain. The microscopy data, which can only be collected on post mortem brains, will help researchers better interpret the MRI data, which can also be collected in living patients.
“It’s going to be a long-term process and it’s the first time that something at this scale has been attempted,” Yendiki explains. “By the end, we’re hoping to have mapped these brain circuits that are implicated in motor and psychiatric disorders at a level of detail previously impossible in the human brain.”
Improving Outcomes for Deep Brain Stimulation Treatments
The findings could improve the outcomes of deep brain stimulation (DBS), a surgical procedure in which electrodes are implanted in the brain. The electrodes are used to apply a corrective electrical current to brain circuits that are malfunctioning in disorders such as Tourette’s, dystonia and obsessive-compulsive disorder.
Because it is a highly invasive procedure, DBS is typically done only after other treatments have proven unsuccessful.
A more precise understanding of the pathways involved in these disorders could enable the more efficient placement of electrodes and provide patients with better symptom relief and quality of life.
The findings could also help with less invasive forms of therapy such as transcranial magnetic stimulation, where an electromagnetic coil is placed on the scalp and transmits magnetic pulses to the brain through the skull.
For Yendiki, digging deeper into these pathways has both a scientific and a personal appeal.
“The images are pretty spectacular, so I like the visual aspect of it and how it combines a lot of different disciplines,” she says. “I like the physics behind capturing the images and the mathematics behind analyzing the data.”
“I also have a personal connection to one of the disorders I will be targeting,” she says, noting that her mother suffered from obsessive compulsive disorder.
“For me, it’s rewarding to be able to make an impact in understanding the brain circuits that are impacted in that disorder in order to help clinicians provide better care for patients.”
Type
Centers and Departments
Publications
Support Research at Mass General
Your gift helps fund groundbreaking research aimed at understanding, treating and preventing human disease.
Check out the Mass General Research Institute blog
Bench Press highlights the groundbreaking research and boundary-pushing scientists working to improve human health and fight disease.